Genetic Characterization of Novel Oral Polio Vaccine Type 2 Viruses During Initial Use Phase Under Emergency Use Listing — Worldwide, March–October 2021
Weekly / June 17, 2022 / 71(24);786–790
Javier Martin, PhD1; Cara C. Burns, PhD2; Jaume Jorba, PhD2; Lester M. Shulman, PhD3; Andrew Macadam, PhD1; Dimitra Klapsa, PhD1; Manasi Majumdar, PhD1; James Bullows, PhD4; Ann Frolov, MS4; Ryan Mate, MS1; Erika Bujaki, MS1; Christina J. Castro, MS, MBA5; Kelley Bullard, MS5; John Konz, PhD6; Kaija Hawes, MBA7; Jillian Gauld, PhD9; Isobel M. Blake, PhD10; Laina D. Mercer, PhD7; Feyrouz Kurji, MPP8; Arie Voorman, PhD9; Ousmane M. Diop, PhD11; M. Steven Oberste, PhD2; John Modlin, MD12; Grace Macklin, PhD11; Martin Eisenhawer, PhD11; Ananda S. Bandyopadhyay, MBBS7; Simona Zipursky, MS11 (View author affiliations)
View suggested citationSummary
What is already known about this topic?
Sabin oral polio vaccine virus can revert to neurovirulence in populations with low immunity. A genetically stable novel type 2 oral poliovirus vaccine (nOPV2) was authorized for outbreak response use under a World Health Organization Emergency Use Listing.
What is added by this report?
Global nOPV2 genomic surveillance during March–October 2021 confirmed genetic stability of the primary attenuating site.
What are the implications for public health practice?
nOPV2 is used to respond to poliovirus outbreaks with comparatively low risk for generating new circulating strains. Given the background immunity, population dynamics, and scale of use, the consistent pattern of genetic characteristics of nOPV2 isolates is encouraging. nOPV2 surveillance should continue for the duration of the Emergency Use Listing.
The emergence and international spread of neurovirulent circulating vaccine-derived polioviruses (cVDPVs) across multiple countries in Africa and Asia in recent years pose a major challenge to the goal of eradicating all forms of polioviruses. Approximately 90% of all cVDPV outbreaks are caused by the type 2 strain of the Sabin vaccine, an oral live, attenuated vaccine; cVDPV outbreaks typically occur in areas of persistently low immunization coverage (1). A novel type 2 oral poliovirus vaccine (nOPV2), produced by genetic modification of the type 2 Sabin vaccine virus genome (2), was developed and evaluated through phase I and phase II clinical trials during 2017–2019. nOPV2 was demonstrated to be safe and well-tolerated, have noninferior immunogenicity, and have superior genetic stability compared with Sabin monovalent type 2 (as measured by preservation of the primary attenuation site [domain V in the 5’ noncoding region] and significantly lower neurovirulence of fecally shed vaccine virus in transgenic mice) (3–5). These findings indicate that nOPV2 could be an important tool in reducing the risk for generating vaccine-derived polioviruses (VDPVs) and the risk for vaccine-associated paralytic poliomyelitis cases. Based on the favorable preclinical and clinical data, and the public health emergency of international concern generated by ongoing endemic wild poliovirus transmission and cVDPV type 2 outbreaks, the World Health Organization authorized nOPV2 for use under the Emergency Use Listing (EUL) pathway in November 2020, allowing for its first use for outbreak response in March 2021 (6). As required by the EUL process, among other EUL obligations, an extensive plan was developed and deployed for obtaining and monitoring nOPV2 isolates detected during acute flaccid paralysis (AFP) surveillance, environmental surveillance, adverse events after immunization surveillance, and targeted surveillance for adverse events of special interest (i.e., prespecified events that have the potential to be causally associated with the vaccine product), during outbreak response, as well as through planned field studies. Under this monitoring framework, data generated from whole-genome sequencing of nOPV2 isolates, alongside other virologic data for isolates from AFP and environmental surveillance systems, are reviewed by the genetic characterization subgroup of an nOPV working group of the Global Polio Eradication Initiative. Global nOPV2 genomic surveillance during March–October 2021 confirmed genetic stability of the primary attenuating site. Sequence data generated through this unprecedented global effort confirm the genetic stability of nOPV2 relative to Sabin 2 and suggest that nOPV2 will be an important tool in the eradication of poliomyelitis. nOPV2 surveillance should continue for the duration of the EUL.
Approximately 111 million doses of nOPV2 were administered worldwide during the initial use phase (March–October 2021). During this period, 128 nOPV2 isolates were detected from stool specimens collected as part of routine AFP surveillance from six countries, and 123 nOPV2 isolates corresponding to 39 distinct environmental surveillance samples were detected from seven countries. Whole-genome sequences were generated for these 251 nOPV2 cell-culture isolates.* Intervals from nOPV2 supplementary immunization activity (SIA) to sample collection ranged from zero to 81 days for AFP samples (mean = 12.5 days; median = 7 days) and from 4 to 67 days for environmental surveillance samples (mean = 22.8; median = 16). Each nOPV2 isolate sequence was compared with that of the nOPV2 vaccine strain (GenBank ID MZ245455), and isolates were classified into one of nine categories, based on their risk profile and loss of key attenuating nOPV2 mutations (Figure 1). Among the 251 isolates, 32 (13%) were classified as category 9 (no changes from nOPV2), and 213 (85%) were classified as category 8, showing no reverting mutations in domain V, no recombination, and 0–5 VP1 substitutions. In addition, six isolates were shown to be recombinant between nOPV2 and Sabin 1 or unidentified species C enteroviruses, with crossover points located in the P3 genomic region (classified as category 6). None of the isolates had changes in the primary attenuation site (domain V) that would be predicted to increase neurovirulence (i.e., no changes that strengthen the stability of the secondary structure of the RNA base-pairing [stem]). The most frequent mutations were noted at nucleotide positions that have been shown or inferred to slightly decrease attenuation when present individually (Supplementary Figure, URL https://stacks.cdc.gov/view/cdc/118054). Individual genomes contained from zero to five of these mutations in different combinations (Table). In some cases, second-site mutations compensating for the effect of such mutations were observed. The number of mutations increased with time after corresponding SIA campaigns (7–9) (Figure 2). A higher frequency of mutations reducing attenuation was observed in nOPV2 isolates from environmental surveillance samples than from AFP isolates. None of these excreted viruses has been tested for neurovirulence using the polio transgenic mouse model, but most are similar to viruses evaluated during fecal shedding in clinical trials, which showed no evidence for reversion in the primary attenuation site (3). In addition, molecular clones constructed to contain increasing numbers of the observed variants have thus far failed to demonstrate neurovirulence similar to or higher than that of Sabin 2 with the A481G reversion alone in transgenic mice, which is observed almost universally within 14 days of replication in Sabin OPV2 recipients (3,5,10) (Andrew Macadam, PhD, et al., National Institute for Biological Standards and Control, personal communication, April 2022).
In addition to nOPV2 isolates, whole-genome sequences of 331 cVDPV2 isolates from outbreaks in countries geographically associated with nOPV2 use were determined. None was found to contain any of the three nOPV2-specific nucleotides in the capsid-coding region at positions 814, 817, and 1,375, suggesting that none of the cVDPV2 isolates sequenced represented an nOPV2-derived recombinant virus.
Discussion
The most consequential risks known to be associated with use of live attenuated Sabin OPVs include the emergence of VDPVs resulting from reversion to neurovirulence, circulation of the vaccine strain in certain population settings, and the rare cases of vaccine-associated paralytic poliomyelitis in vaccine recipients or their close contacts. Since 2016, after cessation of routine use of type 2-containing OPV, the risk for seeding cVDPV2 emergence and spread with use of monovalent Sabin OPV2 in response to cVDPV2 outbreaks in areas of low background immunity has been a concern (1). Although nOPV2 is not expected to eliminate these risks from a biologic perspective, a primary goal of nOPV2 development and deployment was to substantially reduce the risks. Data from preclinical, phase I, and phase II studies with nOPV2 demonstrated the superior genetic stability of the primary attenuating site compared with Sabin OPV2 (3,4). However, given the complexity of evolution of live viruses, interplay with host and environmental factors, and the rarity of some of the outcome indicators of genetic and phenotypic stability, large-scale nOPV2 use in immunization campaigns and corresponding monitoring of genetic stability of isolates from the field presented unique opportunities to further augment understanding of the vaccine’s behavior and its potential public health impact.
Monitoring the genetic characteristics of nOPV2 isolates included identifying nOPV2-specific modifications in the genome and looking for changes that are known to reduce genetic stability and increase neurovirulence. Across 251 isolates analyzed during the period considered for this report, no reversions were detected in the primary attenuation site of nOPV2; this is in striking contrast to Sabin OPV2, which reverts in this site in nearly all vaccinees within a few days of vaccine administration. Mutations altering base pairing in RNA secondary structures in the 5’ nontranslated region were observed, as well as capsid mutations affecting antigenicity and attenuation. However, few, if any, of the mutation combinations identified in nOPV2 isolates would cause the nOPV2 strain to approach the neurovirulence of Sabin 2 with the A481G reversion alone. Some nOPV2 viruses excreted during the initial use phase (March–October 2021) showed more extensive variation than that of viruses observed during clinical trials, as expected from the large number of nOPV2 isolates analyzed, although similar polymorphisms at all relevant sites (including sites at both the 5’ nontranslated and capsid regions) were observed in the clinical trials. The higher frequency of mutations reducing attenuation observed in nOPV2 isolates from environmental surveillance samples was likely due, at least in part, to the longer average intervals between SIAs and sample collection for these samples compared with intervals between SIAs and collection of samples from AFP surveillance. Six nOPV2 isolates were found to be recombinants between nOPV2 and Sabin 1 or a species C enterovirus, resulting in loss of nOPV2 3D polymerase mutations. Such recombination events might increase the chance for further recombination but are not themselves expected to have a substantial effect on virus attenuation.
The findings in this report are subject to at least three limitations. First, time since first use is one of the main factors in OPV evolution. Thus, ongoing monitoring of isolates from the field will be important to confirm or modify the observations noted here. Second, several environmental surveillance samples were associated with multiple nOPV2 isolates, which might have skewed results for frequency and temporal analysis. Adequacy of surveillance in areas of use might have affected the analysis because the source data are dependent on the sensitivity of the AFP and environmental surveillance systems. Finally, triangulation of such analyses with clinical case characteristics, safety data, and other epidemiologic factors will be important to assess impact on disease or outbreak dynamics. Future analyses should focus on spatial and temporal relationship of nOPV2 SIAs with the pattern and impact of polymorphisms in the genome.
Overall, the unprecedented global health effort for field monitoring of nOPV2 use and genomic surveillance of confirmed nOPV2 isolates over the period of initial use under EUL authorization affirmed the genetic stability profile of nOPV2, with the World Health Organization approving wider use under EUL. Given the broad spectrum of background immunity, population dynamics, and scale of use, the consistency in the pattern of genetic characteristics of nOPV2 isolates is a promising trend.
Acknowledgments
Global Polio Laboratory Network laboratories; World Health Organization regional laboratory coordinators; Shahzad Shaukat, World Health Organization headquarters; Rachel Lee Keerthana Raju, nOPV Genetic Characterization Sub-Group; nOPV Working Group; BioFarma.
Corresponding author: Cara C. Burns, zqd1@cdc.gov, 404-639-5499.
1National Institute for Biological Standards and Control, Hertfordshire, United Kingdom; 2Division of Viral Diseases, National Center for Immunization and Respiratory Diseases, CDC; 3McKing Consulting Corporation, Chamblee, Georgia; 4IHRC, Atlanta, Georgia; 5Cherokee Nation Businesses, Catoosa, Oklahoma; 6PATH, Seattle, Washington; 7The Bill and Melinda Gates Foundation, Seattle, Washington; 8FdK Consulting, LLC, London, United Kingdom; 9Institute for Disease Modeling, Global Health, The Bill and Melinda Gates Foundation, Seattle, Washington; 10Medical Research Council Centre for Global Infectious Diseases Analysis, School of Public Health, Imperial College, London, United Kingdom; 11World Health Organization, Geneva, Switzerland; 12Dartmouth University, Hanover, New Hampshire.
All authors have completed and submitted the International Committee of Medical Journal Editors form for disclosure of potential conflicts of interest. Isobel M. Blake, John Konz, Andrew Macadam, Javier Martin, John Modlin, and M. Steven Oberste report institutional support from the Bill and Melinda Gates Foundation. Andrew Macadam reports support for travel to consortium meetings from the Bill and Melinda Gates Foundation and PATH. Lester M. Shulman is an independent consultant for the McKing Consulting Corporation, an unpaid member of the Israel National Certification Committee for Polio, and a volunteer member of the Emergency Response Team for the current cVDPV3 polio outbreak in Israel. No other potential conflicts of interest were reported.
* Sequences deposited in GenBank.
References
- Alleman MM, Jorba J, Henderson E, et al. Update on vaccine-derived poliovirus outbreaks—worldwide, January 2020–June 2021. MMWR Morb Mortal Wkly Rep 2021;70:1691–9. https://doi.org/10.15585/mmwr.mm7049a1 PMID:34882653
- Yeh MT, Bujaki E, Dolan PT, et al. Engineering the live-attenuated polio vaccine to prevent reversion to virulence. Cell Host Microbe 2020;27:736–751.e8. https://doi.org/10.1016/j.chom.2020.04.003 PMID:32330425
- Wahid R, Mercer L, Macadam A, et al. Assessment of genetic changes and neurovirulence of shed Sabin and novel type 2 oral polio vaccine viruses. NPJ Vaccines 2021;6:94. https://doi.org/10.1038/s41541-021-00355-y PMID:34326330
- De Coster I, Leroux-Roels I, Bandyopadhyay AS, et al. Safety and immunogenicity of two novel type 2 oral poliovirus vaccine candidates compared with a monovalent type 2 oral poliovirus vaccine in healthy adults: two clinical trials. Lancet 2021;397:39–50. https://doi.org/10.1016/S0140-6736(20)32541-1 PMID:33308429
- Wahid R, Mercer L, Gast C, et al. Evaluating stability of attenuated Sabin and two novel type 2 oral poliovirus vaccines in children. NPJ Vaccines 2022;7:19. https://doi.org/10.1038/s41541-022-00437-5 PMID:35149714
- Macklin G, Peak C, Eisenhawer M, et al.; nOPV2 Working Group. Enabling accelerated vaccine roll-out for public health emergencies of international concern (PHEICs): novel oral polio vaccine type 2 (nOPV2) experience. Vaccine 2022;S0264-410X(22)00195-5. https://doi.org/10.1016/j.vaccine.2022.02.050 PMID:35307230
- World Health Organization. Polio laboratory manual. 4th ed. Geneva, Switzerland: World Health Organization; 2004. https://apps.who.int/iris/handle/10665/68762
- Majumdar M, Klapsa D, Wilton T, et al. Isolation of vaccine-like poliovirus strains in sewage samples from the United Kingdom. J Infect Dis 2018;217:1222–30. https://doi.org/10.1093/infdis/jix667 PMID:29309594
- Montmayeur AM, Ng TF, Schmidt A, et al. High-throughput next-generation sequencing of polioviruses. J Clin Microbiol 2017;55:606–15. https://doi.org/10.1128/JCM.02121-16 PMID:27927929
- Konz JO, Schofield T, Carlyle S, et al. Evaluation and validation of next-generation sequencing to support lot release for a novel type 2 oral poliovirus vaccine. Vaccine X 2021;8:100102. https://doi.org/10.1016/j.jvacx.2021.100102
FIGURE 1. Classification of novel oral poliovirus vaccine isolates into nine categories* based on genome sequence composition — worldwide, March–October 2021
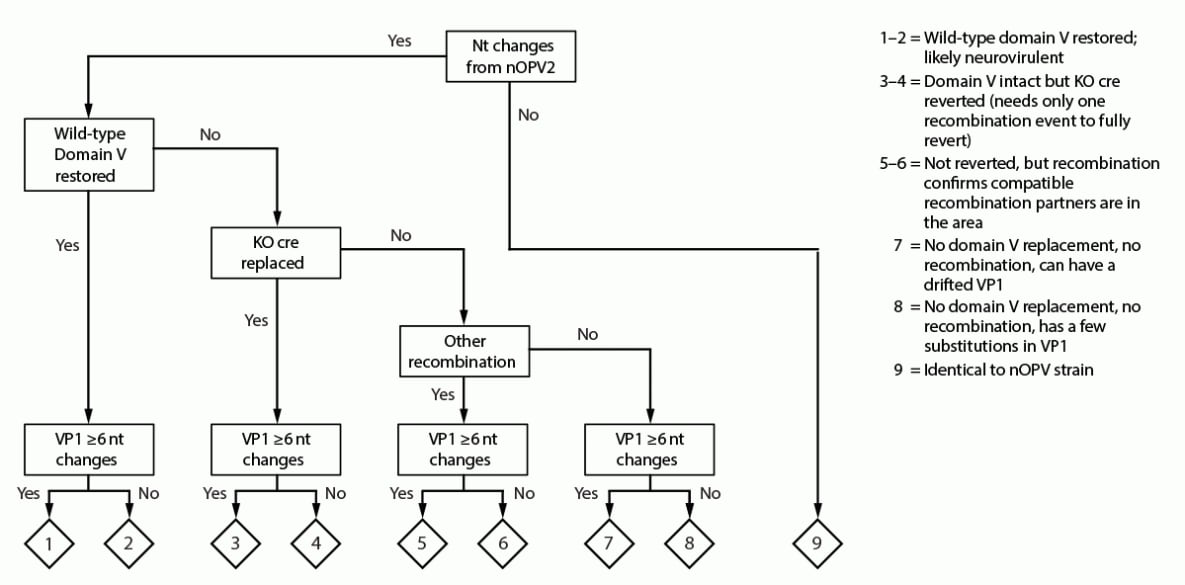
Abbreviations: KO cre = knockout of cis-acting replication element; nOPV2 = novel oral poliovirus vaccine type 2; nt = nucleotide; VP1 = viral capsid protein 1.
* Category groups are ranked according to level of concern based on expected neurovirulence, with 1 being the highest and 9 being the lowest.
* These isolates also contained mutation G3425A (VP1-E295K), which counteracts the effect of VP1-N171D.
† One of these isolates also contained mutation C550U, likely increasing attenuation slightly.
§ One isolate also contained mutation G3425A (VP1-E295Q), which counteracts the effect of VP1-N171D and mutation C392U, likely increasing attenuation slightly. The second isolate also contained mutation A129G, likely increasing attenuation slightly.
¶ This isolate also contains mutation G139A, likely increasing attenuation slightly.
FIGURE 2. Numbers of total mutations (A), viral protein 1 mutations (B), mutations reducing attenuation (C), and percentage of novel type 2 oral poliovirus vaccine isolates with mutations (D)* found in consensus sequences† through acute flaccid paralysis surveillance and environmental surveillance, after supplementary immunization activities — worldwide, March—October 2021
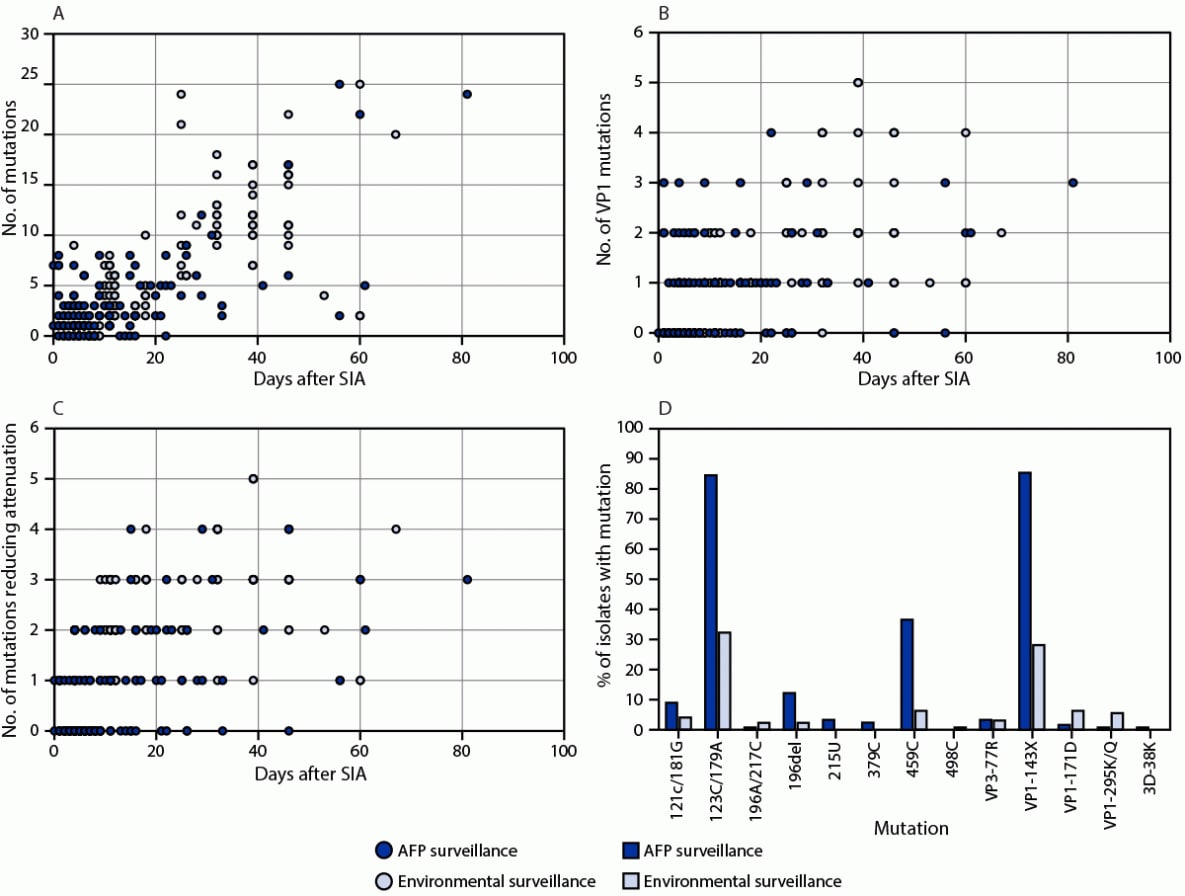
Abbreviations: AFP = acute flaccid paralysis; nOPV2 = novel type 2 oral poliovirus vaccine; SIA = supplementary immunization activity; VP1 = viral protein 1.
* Mutations that have been shown/inferred to decrease attenuation (121C/181G, 123C/179A, 196A/217C, 196del, 215U, 379C, 459C, 498C, VP1-143X, and VP1-171D), antigenic mutations VP3-77R and VP1-295K/Q, and reversion mutation 3D-38K.
† Whole-genome sequencing from viral RNA extracted from culture-amplified virus directly from culture or from Flinders Technology Associates cards spotted with culture supernatant was performed using next generation sequencing methods using a combination of random and poliovirus-specific primers to ensure whole-genome coverage. https://apps.who.int/iris/handle/10665/68762
Suggested citation for this article: Martin J, Burns CC, Jorba J, et al. Genetic Characterization of Novel Oral Polio Vaccine Type 2 Viruses During Initial Use Phase Under Emergency Use Listing — Worldwide, March–October 2021. MMWR Morb Mortal Wkly Rep 2022;71:786–790. DOI: http://dx.doi.org/10.15585/mmwr.mm7124a2.
MMWR and Morbidity and Mortality Weekly Report are service marks of the U.S. Department of Health and Human Services.
Use of trade names and commercial sources is for identification only and does not imply endorsement by the U.S. Department of
Health and Human Services.
References to non-CDC sites on the Internet are
provided as a service to MMWR readers and do not constitute or imply
endorsement of these organizations or their programs by CDC or the U.S.
Department of Health and Human Services. CDC is not responsible for the content
of pages found at these sites. URL addresses listed in MMWR were current as of
the date of publication.
All HTML versions of MMWR articles are generated from final proofs through an automated process. This conversion might result in character translation or format errors in the HTML version. Users are referred to the electronic PDF version (https://www.cdc.gov/mmwr) and/or the original MMWR paper copy for printable versions of official text, figures, and tables.
Questions or messages regarding errors in formatting should be addressed to mmwrq@cdc.gov.