Basic Tutorial on Wireless Communication and Electronic Tracking: Technology Overview
1.0 Introduction
The Mine Improvement and New Emergency Response Act of 2006 (MINER Act) requires mine operators to adopt underground communications and electronic tracking (CT) systems that meet specific performance goals. The MINER Act was signed into law on June 15, 2006. It amends the Federal Mine Safety and Health Act of 1977 and is intended to improve the safety of miners. Among other things, it provides updated requirements for emergency response, incident command and control, mine rescue teams, and incident notification.
The MINER Act provides two sets of requirements for communications and tracking:
- The first set of requirements must be implemented by coal mines no later than 60 days after enactment of the MINER Act. It requires the mine owners to submit an emergency response plan (ERP) to be submitted that provides for a redundant means of communication with the surface for underground workers, such as a secondary telephone or equivalent two-way communication. The ERP also must provide for above-ground personnel to be able to determine the current, or immediately pre-accident, location of all underground personnel. Any system so utilized must be functional, reliable, and calculated to remain serviceable in a post-accident setting.
- The second set of requirements must be implemented by coal mines no later than three years after enactment of the MINER Act (June 15, 2009). It requires a plan to be submitted for approval that provides for post-accident communication between underground and surface personnel via a wireless two-way medium, and an electronic tracking system that permits surface personnel to determine the location of any persons trapped underground or set forth within the plan the reasons such provisions cannot be adopted. Where such plan sets forth the reasons such provisions cannot be adopted, the plan must set forth the operator’s alternative means of compliance. Such an alternative must approximate, as closely as possible, the degree of functional utility and safety protection provided by the wireless two-way medium and electronic tracking system.
One of the goals of the MINER Act is to provide wireless communications and location information between underground workers and surface personnel following an underground accident. The CT technology that is available, or anticipated to be available soon, to meet these goals may be unfamiliar to the mining professionals who need to purchase or use this technology. The purpose of this tutorial is to introduce the different types of CT technologies, describe how they work, and provide guidelines that allow the reader to evaluate and compare systems.
This tutorial provides a brief overview of CT systems intended to meet the needs of people who require a basic understanding of the distinguishing features and operations of different CT systems. The readers of this tutorial are assumed to be associated with coal mining, and therefore familiar with coal mining operations and terminology. After reading this tutorial the reader should walk away with an adequate understanding of CT technologies that are being proposed for use in underground coal mines. Future tutorials will address those aspects of CT systems for people with a technical background who need information to compare systems and whose job responsibilities require advanced knowledge. For example, the mine’s communications expert will need to understand how the underground environment influences performance of CT systems, how different CT systems work, and advantages and disadvantage of different CT systems.
2.0 CT Technology Overview
This section provides a brief overview of the technologies proposed for wireless communications and electronic tracking systems in underground coal mines.
The term system describes a collection of components that must be connected or operated together to make a working arrangement - in this case a communications system or a tracking system. A mine may have a communications system that is completely separate from the tracking system. An integrated system is a single system that provides both communications and tracking. Communications systems and electronic tracking systems have similar principles of operation, but because they address different needs, they are best understood if discussed separately. Therefore, this chapter breaks CT systems into five parts: Communications Basics, Communications Systems Principles of Operations, Tracking Systems Principles of Operations, Network Options, and the Mine Operations Center.
2.1 Communications Basics
All modern communications and electronic tracking (CT) systems depend on the transmission and reception of energy. Electromagnetic energy is energy associated with nearly all modern CT systems, and for all of the systems discussed in this tutorial. Electromagnetic energy is everywhere in the environment and examples include radio waves, visible light, and x-rays. Many common devices depend upon sending or receiving electromagnetic energy for their operation. Some examples are televisions and radios, cell phones, automatic garage door openers, and remote keyless entry fobs for cars. Electromagnetic energy can be visualized as a traveling wave of electric and magnetic energy. All waves are characterized by their wavelength and amplitude.
The wavelength is the distance between adjacent peaks of a wave. Notice that the wavelength of the dashed line wave in Figure 2-1 is longer than that of the solid line wave. The unit of measure for wavelength is meters or feet. The amplitude is a measure of how tall the wave is. The dashed line wave in Figure 2-1 has greater amplitude than the solid line wave.
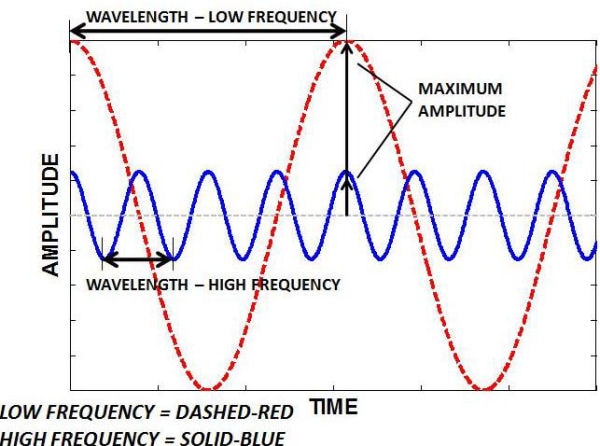
Figure 2-1. The Main Characteristics of Waves
In discussing their CT systems, manufacturers will typically mention the frequency at which the systems operate. The frequency relates directly to the wavelength. Frequency is a measure of the number of up and down oscillations or repetitions of the wave over a fixed length of time. The fewer oscillations that a wave has within a fixed period, the lower the resulting frequency will be (and the longer the wavelength). The dashed wave in Figure 2-1 has fewer oscillations than the solid wave, so it has a lower frequency. Cycles per second, or Hertz (Hz), is the measure of frequency. Low frequency waves have longer wavelengths while high frequency waves have shorter wavelengths. The maximum amplitude of a wave is the peak of the wave, labeled in Figure 2-1, whereas the amplitude of a wave is its value at any time, labeled on the y-axis.
The frequency (or wavelength) of a particular CT system is a very important factor in its design and operation because certain wavelengths lend themselves well to travelling through a given transmission media. For instance, very long wavelengths can travel a significant distance through the earth. Radio communications use short wavelengths, which travel well through the air or down tunnels, while extremely short wavelengths are required to travel within fiber optic cables.
This fundamental background in electromagnetic energy leads us to the principles of operation behind communications systems.
2.1.1 Physical Communications Links
Communications technology involves electronic devices that allow people to talk or send information to each other. In its most elementary form, a communications system is made up of a transmitter, transmission medium, and a receiver. Figure 2-2 shows these basic components. The transmitter is the device that sends out the signal, and the signal contains the information in the form of an electromagnetic wave. The information could be part of a conversation or a text message. The signal travels through or along a transmission medium such as air, wires, metallic pipes, fiber optic cable, or even the ground. A receiver then picks up the signal and a physical communication link is accomplished.
In the example shown in Figure 2-2, there is only one transmitter and receiver pair involved in the communications path, or one physical communication link. Obviously, for two people to talk back and forth a transmitter and receiver are required on both ends of the communication. A transceiver is a device that combines the transmitter and receiver into one unit; a walkie-talkie is a good of example of such a device.
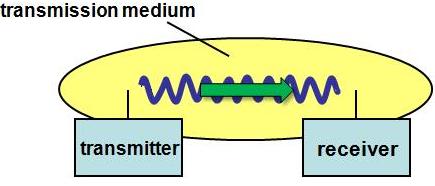
Figure 2-2. The Basic Components of a Physical Communications Link
The requirements of most communications systems are more complex than that depicted in Figure 2-2 because multiple physical communications links may be involved in establishing the connection between the sender and receiver. For example, a cell phone may transmit over the air to a cell tower, which transfers the signal to a telephone line, and then to a different cell tower, and finally over the air to another cell phone. The connection between the sender and the receiver involves multiple physical communication links from the user’s phone, to the cell tower, to the phone line, through the telephone system, back to another cell tower, and finally to the receiver’s phone. These multiple link systems are called networks.
2.1.2 Networks
Two people using walkie-talkies to communicate will find that as they increase their separation distance, eventually the distance becomes large enough that they can no longer communicate. This limitation established by the separation distance, or range, occurs because energy is being lost within the transmission media. The transmission media dissipate energy until the energy is so low that the receiver can no longer “hear” the transmitter.
Another factor limiting the range between two transceivers is noise. Noise is unwanted electromagnetic energy that makes it difficult for the receiver to “hear” the transmitter. This is similar to attempting to communicate with someone across a crowded, noisy auditorium. To be heard a person may have to shout (increase power) or get the audience to be quiet (lower the noise level).
Whether the cause is dissipation of energy in the transmission media, noise, or a combination of both, eventually the range of a single physical communication link becomes limited. Adding a node between the transceivers can increase the allowable distance between them. Among other functions, the node acts as a repeater, which relays the message from one transceiver to the next (in either direction) by automatically retransmitting the signals it receives. This retransmission may also involve converting the transmission frequency so that it can transmit across a different transmission medium, such as a wire. The retransmission may also involve sending the signal to multiple destinations or amplifying the signal. With the additional device of a node in the communications link, the result is a simple communications network. A communications network is a system of interconnected pieces of communications equipment used to transmit or receive information.
Figure 2-3 shows a simple network with two physical communications links: one from the radio to the node (repeater) on the left, and the other from the repeater to the radio on the right. Generally, the same antenna on the node receives and retransmits the message, but there may be separate antennas dedicated to each function. In Figure 2-3, the node receives the radio frequency (RF) signal from the radio on the left. The signal travels into the node where internal electronics process the signal, amplify it, and then retransmit it. The radio on the right then receives the signal. In this case, the situation is more complicated than simply connecting two radios by an air medium.
It is easy to imagine extending the range of the communications shown in Figure 2-3 even further by adding an additional repeater between the two radios. Figure 2-4 shows two nodes, each with a defined communications range. The dotted line shows the range of the node on the left and the solid line shows the range of the node on the right. The antennas of the nodes are within the range of each other, because the left node’s antenna is within the solid oval and the right node’s antenna is within the dotted oval. Because the nodes are within each other’s range, they can pass communications in either direction between the two nodes. With this network, two radios within range of either node will be able to communicate with each other.
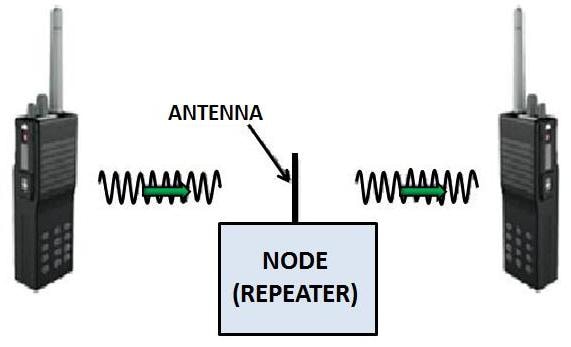
Figure 2-3. Example of a Very Simple Network
The communications path between two radios can be referred to as direct point-to-point (involving only one physical communication link), or it can be achieved through a complex network that connects the source and destination (involving multiple physical communications links). The interconnection between the nodes in the network can be wireless or wired. Fiber-optic cable or other means can also be used to connect the nodes. Figure 2-5 shows three examples of network configurations for interconnected nodes.
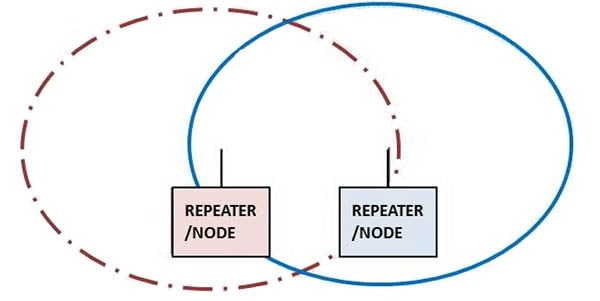
Figure 2-4. The Communications Range of Two Nodes
The solid lines in the above diagrams (Figure 2-5) represent physical communications links between the nodes. A well-designed network configuration increases the survivability of communications (the potential for the system to continue operating after an accident) should one or more of the nodes fail. For example, if one of the nodes in the ring configuration fails, the remaining communications can survive by reversing the direction of traffic in the region of the failed node. In the star configuration, the failure of an outlying node does not disrupt the rest of the network, but the failure of the center node will shut down the entire network.
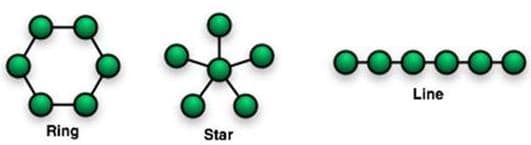
Figure 2-5. Examples of Network Configurations
2.1.3 Wireless Versus Wired Systems
The definition of the term wireless as used in this document, and as it relates to underground mines, requires some discussion here. Most people consider a cell phone to be a wireless device, but many cell phone calls actually travel over conventional telephone lines before reaching the receiving party’s cell phone. Neither person recognizes the use of conventional telephone lines during the communication. The main convenience for the user is that there are no wires or cables connected to the handheld device, even though they are integrally involved in the signal transmission. This example of “wireless” communications is consistent with the definition adopted for this tutorial: “a system that operates locally without wires” (see the Glossary for more details).
Two people using walkie-talkies that can communicate over significant distances exemplify another possible definition of wireless. In this case, there is only one type of physical communications link. It is common to call such communications devices radios, and to refer to the communication between these radios as wireless. In relation to mining applications, some suggest that the definition of wireless should be restricted to the simple concept of “one physical link” (no intervening cables, nodes, or devices of any kind). Another definition of wireless commonly used is that the system can contain multiple physical communications links but that each link has to be wireless.
There has been considerable debate over exactly what Congress intended in the MINER Act by requiring “wireless” communications via “a wireless two-way medium.” To address this issue within the context of this tutorial, two assumptions were made: First, for practical purposes, the communications system miners use must be an untethered device (i.e., one without connecting wires or cables). An untethered device, such as a small radio, can be worn or carried by the miner. Second, the principal intent is to ensure a survivable connection between the miner and the surface throughout the area where the miner may need to work or travel. Within this tutorial, a wireless communications system is defined as one that does not require a physical connection to the miner’s handheld radio. Therefore, in this context, wireless refers to any system in which the miner uses an untethered device for communications. Readers should review the latest guidance from the Mine Safety & Health Administration (MSHA), and state regulatory agencies, for their definition of wireless and detailed requirements for compliance with the MINER Act.
2.1.4 Primary Versus Secondary Communication Systems
The communications technologies can be classified into two main categories, primary communication systems or secondary communications systems, which are explained in more detail below.
Primary communications systems are characterized as those that have a transceiver small enough to be comfortably worn (carried) by a miner throughout an entire shift. Primary communications systems typically function at conventional radio communications frequencies, use small antennas, have a long battery life, and provide sufficient radio channels for general operations. Of the systems that will be discussed in this tutorial, the leaky feeder and node-based systems are considered primary systems.
Secondary communications systems require a larger and heavier antenna, making these systems still portable, but less manageable for wearing throughout a shift. Secondary communications systems typically operate at lower, non-conventional radio frequencies and generally have only a single channel for communications, and do not have sufficient throughput capacity for general operations. Of the systems discussed in this tutorial, the medium frequency (MF) and through-the-earth (TTE) systems are considered secondary communications systems.
2.2 Communications Systems Principles of Operation
2.2.1 Hardwired Systems
Many underground coal mines use some form of telephone as the primary means of communications between the surface and the underground miners. It is easy to imagine two phones (or transceivers, as introduced in Section 2.1.1) directly connected by wires to form the physical communications link. Relating to the previous discussion of Figure 2-2 in Section 2.1.1, the energy from the transmitter directly couples into the transmission medium, which in this case is a wire or cable. For mine pager phones, which are the most common form of communications in underground mines, two wires are typically used. Connecting additional phones into the same wires forms a network of phones. With this network configuration, the phones operate in a page mode in which all the telephones broadcast simultaneously when a button is pressed on the transmitting pager phone. The system works well in the case of an emergency when all miners must be notified. However, there is no capability for private or simultaneous conversations.
Some mines use a dial telephone system similar to a commercial phone system, but the mine phones are completely separate from, and cannot communicate with, a commercial phone system. Private conversations between miners are possible. Key personnel are assigned ring codes to indicate when the phone is for them. Personnel must also remember the phone numbers of other workers with whom they wish to talk.
Another type of hardwired communications system is the trolley wire or carrier phone. Mines with extensive rail haulage use trolley phones. The electromagnetic (EM) signal couples to the trolley power line. The physical communications link is similar to the dial telephone described above, except the wire is the trolley power line. Phone locations include the trolley-powered vehicles and strategic areas that identifiable in the mine. Communications to cages and elevators in vertical and slope shafts use the same system.
Despite their capabilities, hardwired communications systems are generally not robust. The typical network configuration for a hardwired phone system is the bus structure shown in Figure 2-6. The connecting wires are easily broken or shorted by rock falls. Once a line is shorted, the communications may be severely affected or cease altogether. These systems lack redundancy, which is the ability of the network to maintain communications with the surface even when there is a disruption of a single pathway. In an underground coal mine the available network configurations are highly limited. The long, linear tunnels and the limited access pathways to the surface restrict the design choices.
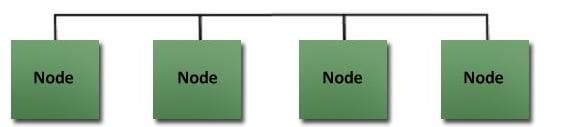
2.2.2 Wireless Systems
The following sections present four different wireless communications technologies. The primary difference between them is their frequency bands of operation. Each of the frequency bands uses a different mechanism for the propagation of the EM waves. Each of these systems has the advantage of permitting the miner’s radio to be untethered, as discussed in Section 2.1.3.
As shown in Figure 2-7, in a wireless communications link the antennas couple the EM energy from the transmitter to the transmission medium and capture the energy at the receiver location from the medium. The coverage range is the maximum distance between the transmitter and receiver while still maintaining good quality communications. The coverage area is the area within which radio communication is possible.
There are two broad categories of antenna systems - discrete antenna (single-point) systems and distributed antenna (multipoint) systems. Most people are familiar with discrete antenna systems, which are often made of simple pieces of wire. Examples of discrete antennas are TV antennas on a house or the radio antenna on a car. Sometimes the wire is contained in some sort of enclosure for protection, such as the rubber or plastic antenna protectors found on portable radios and cell phones.
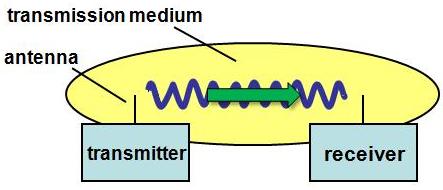
Figure 2-7. Basic Components of Wireless Communications Link
There are two types of discrete antennas - directional and non-directional. A non-directional antenna (sometimes called an omnidirectional antenna) radiates energy more or less uniformly in all directions. This is analogous to how a lighted match or a light bulb radiates light in all directions. On the other hand, a directional antenna focuses the energy in one direction. Using the light analogy, a directional antenna is similar to a flashlight. A flashlight points most of the light energy in one direction. A flashlight uses a reflector (behind the bulb) that is shaped to focus the energy in the desired direction. Similarly, reflectors behind an EM energy source create highly directional antennas, such as in a satellite dish.
In most discrete antenna systems, one antenna both transmits and receives from the transceiver. However, some systems use separate antennas for the transmitting and receiving functions. A discrete antenna has a limited and localized physical size, and the dimensions of the antenna are much smaller than the coverage area or coverage range.
The other broad category of antenna systems is the distributed antenna system (DAS). Unlike a discrete antenna system, where the energy transmits and receives at one location, a DAS distributes the energy over a broad area and the antenna system can be quite large. The use of DAS has been popular for years in providing radio coverage in confined spaces such as tunnels. More recently, DAS systems have been used to provide cellular radio coverage inside large buildings and other hard-to-reach areas, such as parking garages and casinos. Given this history, DAS systems are good candidates for applications in underground mining.
Most DAS applications create a continuous coverage area by providing many overlapping radiation points or continuous radiation along the length of the system. The leaky feeder system discussed in the next section is an example of a DAS that has continuous radiation along the length of the system.
An important limitation of a DAS is that parts of the system require power to boost or amplify the signal. As the signal travels along the length of the DAS, energy is lost due to the energy radiated along the length. To offset this loss, electrical power is required for the amplifiers within the DAS to boost the signal. The availability of power and the safe handling of this power is a significant limiting factor in using a DAS in an underground coal mine.
Another limitation to a DAS system is that it receives the signal along its entire length; therefore, it is also receiving noise over its entire length. As discussed earlier, in Section 2.1.2, noise can reduce the coverage range of a system; therefore, the cumulative noise can limit the size of a DAS.
2.2.2.1 Leaky Feeder Systems
A leaky feeder system used in an underground coal mine typically involves a single large transceiver on the surface that can communicate with all miners’ radios along the length of the system. The transceiver on the surface, called a base station , connects to a DAS system. Leaky feeder systems operate at a frequency that is conventionally used by two-way voice radio communications, with the electromagnetic energy transmitted and received through radio frequencies (RF). Figure 2-8 shows the main components of a leaky feeder communications system.
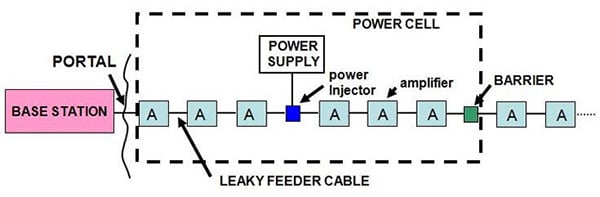
Figure 2-8. A Leaky Feeder Communications System
A DAS consists of a specially designed coaxial cable (commonly called leaky feeder cable) and amplifiers. This leaky feeder cable “leaks” the radio signal in or out along its length, thus creating a continuous coverage area along the tunnels in which the cable is strung. The coaxial cable has regular openings in the outer shield, as shown in Figure 2-9, which permit RF energy to enter or leave the cable. It can receive and transmit signals down its entire length. Wherever a mine desires communications, it installs leaky feeder cable down the entries to the mine. In addition to transporting the RF signal, the center conductor of the cable also carries the DC power (typically 12 volts) for the amplifiers.
Leaky feeder systems for coal mines are commonly marketed as very high frequency (VHF), operating around 150 megahertz (MHz), or one million cycles per second, or ultra high frequency (UHF), operating around 450 MHz. At these operating frequencies, the handheld radios can establish a physical communications link through air, but the range is very limited underground. The leaky feeder system overcomes this range limitation by extending the receiver antenna (the leaky feeder cable) to the general area of the handheld radio, which greatly extends the range and permits surface personnel to talk with distant underground miners. The radio transmits the RF signal, which the leaky feeder cable receives if the radio is within range. The signal travels down the cable, radiating as it travels. If the receiving radio is within RF range of the cable, it receives the signal and makes the connection. Figure 2-10 shows a cutaway view of an underground room-and-pillar coal mine with a leaky feeder cable installed down one entry. The orange dots (dots along the labeled leaky feeder) represent the path of the RF signal.
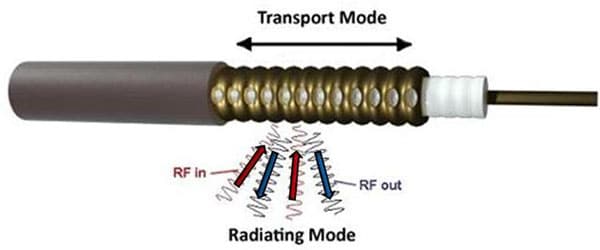
Figure 2-9. Example of a Leaky Feeder Coaxial Cable
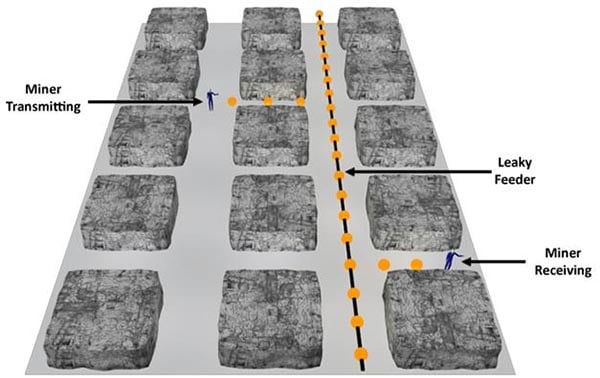
Figure 2-10. Example of a Leaky Feeder Communications System
Leaky feeder cables cannot transport radio signals for indefinite distances. Attenuation (signal power loss) exists in the cable itself, and continual radiation of RF energy flows through the openings in the outer shield. Therefore, a mechanism that periodically boosts the strength of the RF signal is required. Amplifiers are electronic components periodically inserted in the cable to boost the signal by increasing its amplitude. The amplifiers receive their power from a power supply through the center conductor of the cable. For underground coal mine applications, one power supply can generally support six amplifiers. The power supply, amplifiers, and sections of leaky feeder cable form a building block, called a power cell, for the leaky feeder system. Figure 2-11 shows the power cell components of a leaky feeder system. In an underground coal mine, a typical power cell might be 8,700 feet in length.
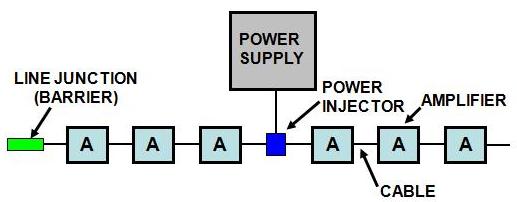
Figure 2-11. Leaky Feeder Power Cell Structure
In a long entry, multiple cells are used to provide radio communications coverage everywhere along the entry. To establish interconnections between power cells, leaky feeder networks typically use a tree configuration as shown in Figure 2-12. The circles are the power cells of the leaky feeder system. The cell furthest to the right is either on the surface or just inside the portal and provides the communications connection to the mine operations center. Should something happen to that power cell, communications with the surface are lost. Therefore, to increase the network’s survivability, some leaky feeders use an alternate communications path to the surface, perhaps through another portal or a borehole to the surface. For the communications link at the surface, ordinary conductors, fiber optic cable, or through-the-air transmission can be used to complete the connection to the mine operations center.
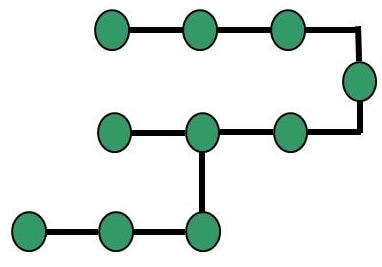
Figure 2-12. A Tree Configuration of Power Cells
2.2.2.2 Node-Based System
Node-based systems refer to systems that use discrete antennas connected to small transceivers called “nodes.” The nodes also contain small computers (microprocessors) that perform a variety of functions. In all node-based systems, the node can detect when a miner’s radio is in range and provides an automatic connection to the network. Beyond that basic function, the capabilities of nodes vary greatly depending on the manufacturer and choice of technology.
In node-based systems, the access link is the first link, which is through the air from the miner’s handheld radio to a node. The access node is the node providing the communications service or link to the miner's radio. The backhaul is the communications path from the access node to the surface. The backhaul links are the connections between nodes - through wires, the air, or both; thus, node-based systems come in many forms.
Node-based communications systems for coal mines can be assembled from a number of different technologies. Wireless Fidelity (Wi-Fi), also referred to as wireless local area network (WLAN), is the foundation of one node-based system used for underground coal mines. Common uses for Wi-Fi systems are in the home, the local coffee shop, and airports to provide wireless access for a computer to the internet or any device or network that uses standards-based Internet Protocol (IP). The advantage of these systems is that many devices and networks support IP, offering a variety of potential applications such as video monitoring or remote control via the internet. In these systems, the access nodes need to communicate with a gateway node located at the mine operations center, which provides the communications link to the surface facilities and supplies message routing information and other data to the access node. The backhaul link is from the access node to the gateway node through wires, fiber, or other radio links. Some proprietary variations of Wi-Fi systems use the wireless link between nodes as alternate backhaul links.
Using UHF radios is another approach to node-based communications. In the underground coal mine environment, UHF radios can communicate directly with each other over significant distances, perhaps 1,000 feet. To extend the communications range, it is necessary to use repeaters (also called nodes) as intermediate components in the communications route. Section 2.1.2 introduced the concept of a node as a device that relays an RF message from one device to the next by automatically retransmitting the signals it receives. Figure 2-13 shows a wireless link between two radios that involves two nodes to complete the connection.
The communications connection between the sender and the receiver is generally from the transmitter to air, air to node, node to node to node (i.e. may possibly involve multiple nodes), and, finally, node to air to receiver. Note that the node-to-node link is also through the air. The manner in which a UHF radio wave travels through the air in a coal mine is different from through the air on the surface. In an underground mine, the tunnel opening guides the UHF waves, which bounce off the walls, floor, and roof. The tunnel acts as a guide, or pipe, for transporting the radio waves. This guiding effect is important because it contributes to a loss of signal power in the RF link through the air, which determines the effective range of the communications link.
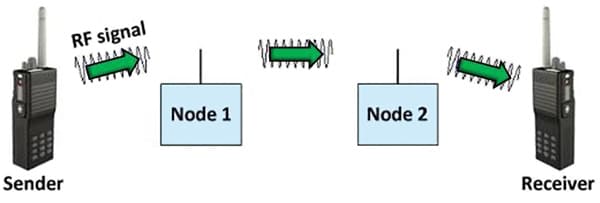
Figure 2-13. A Wireless Route Using Two Intermediate Nodes
The number of nodes participating in the connection between the sender and receiver will depend on the locations of the radios and the nodes and the path or route taken through the nodes to link the radios. Figure 2-14 shows six nodes (numbered orange dots) in a portion of a mine. The route from the sender to the receiver could be through nodes 1-2-3-6, or 1-4-5-6, or one of several other possible combinations of nodes.
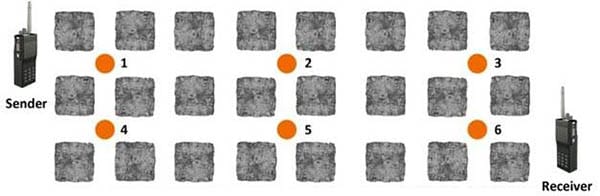
Figure 2-14. Node (Orange Dots) Based Communications System
For a UHF network, fiber-optic cables or metallic conductors can connect the nodes in the entries to the mine. However, because the UHF nodes both receive and transmit UHF signals, a wireless connection is possible, with no cabling needed.
In addition, each node can contain a small computer programmed to detect when another node is within RF range and that node’s identity. The computer can automatically establish a wireless connection between the nodes. When a sender and receiver wish to link, the computers work in concert to determine the optimum route between the participating nodes.
Figure 2-15 shows a cutaway view of a mine with a UHF node-based communications system. Orange dots indicate the RF signal route between the two miners. Should an incident occur that disables one of the nodes, it is possible for the node computers to recognize the loss and to determine a new route for reestablishing the communications link, as shown in Figure 2-16.
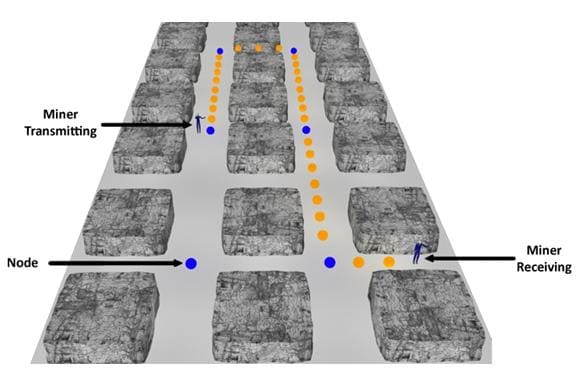
Figure 2-15. UHF Node-Based Communications System
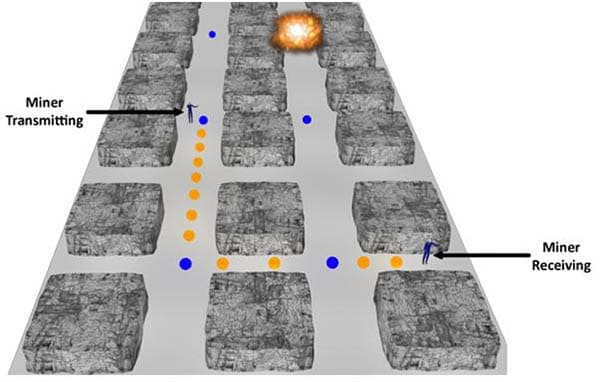
Figure 2-16. Node-Based Network Establishes Alternate Communications Path
As demonstrated, a UHF node-based communications system can be made to be quite robust (i.e., able to reestablish or reconfigure itself following an accident). To provide an alternate communications path following an accident, two requirements must exist: (1) There must be enough nodes within RF range of each other to establish alternate routes when required. This requirement is partly a cost issue; additional nodes cost additional money. (2) The nodes must be capable of automatically reconfiguring the network. This means that the manufacturer must install the computer chips and appropriate programming into the nodes.
The type of node-based network described above, using UHF radios as an example, is a mesh network. There are a variety of mesh network types. A mesh network where all the nodes, including the miner’s radio, can relay the network traffic and automatically reconfigure the network over any arbitrary route in real time is an ad hoc mesh network. A mesh network that can only reconfigure periodically is a constrained mesh, e.g., one where the reconfiguration is dependent on predefined alternate routes, or one in which the miner’s device cannot relay traffic. A full mesh network is a network in which each node connects to every other node by a direct physical communication link. A partial mesh network is a network where each node connects to several other nodes, but not to all nodes. Because room-and-pillar mines can extend for miles, a full mesh may be impossible. The nodes in Figures 2-15 and 2-16 are able to communicate with the adjacent outby and inby nodes, as well as the adjacent node in the crosscut. These nodes form a partial mesh network.
2.2.2.3 Medium Frequency System
Medium frequency (MF) communications systems typically operate at around 500 kilohertz (kHz - 1,000 cycles per second). In addition to their operating frequency, what distinguishes them from other communications systems is the way the RF signals travel in the mine. At these frequencies, the radio signals couple onto metallic conductors such as power lines, phone lines, wire lifelines, other electrical wiring, and metal pipes. The conductors play the same role as the coaxial cable does in the leaky feeder systems, as conduits for the radio signal. MF radio signals travel along the conductor. In addition, the conductor acts as a distributed antenna, able to transmit and receive signals continuously along its length, just like the leaky feeder cable.
Direct MF radio to MF radio communication distance is very limited unless metal conductors are present along the communications path. Since many mine entries already have conductors and because installing simple conductors is inexpensive, the MF communications distance easily extends to miles without the need for a repeater or amplifier. Figure 2-17 shows a typical connection between two MF radios.
MF radios and antennas are considerably heavier and larger than UHF or VHF handheld radios. Therefore, it is not likely that the miner would continuously carry these devices while performing daily work. The MF radio might be used as a redundant communications system or a system used mainly for emergencies.
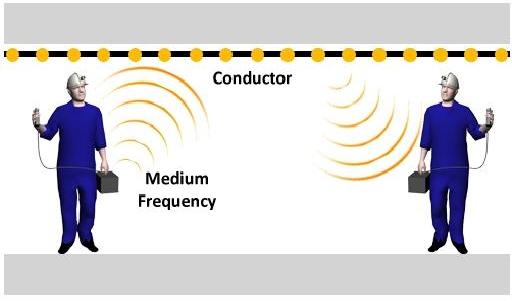
Figure 2-17. Typical Connection between Two MF Radios
An up/down frequency converter is able to interconnect MF and higher frequency systems like UHF or VHF. The device changes RF signals received in one frequency band to RF signals transmitted in a higher or lower frequency band. For example, the converter can down-convert signals received in UHF to signals transmitted at MF, or conversely, receive MF and transmit UHF. Thus, the converter is somewhat like a repeater, except that it retransmits the signal it receives at a different frequency. These up/down frequency converters are referred to as bridge repeaters or bridge nodes.
The bridge repeaters provide flexibility in networking by permitting hybrid systems, i.e. systems that combine multiple frequency technologies. Figure 2-18 shows a sample hybrid configuration. A miner, using a UHF handheld radio, can transmit to a bridge repeater that receives the UHF and retransmits MF, which, in turn, couples to a conductor. The conductor transmits and receives MF as the signal travels along. At some point, another bridge repeater picks up the MF signal which then transmits the message in UHF. Another miner with a UHF handheld radio can receive the message. The receiving miner can send a reply which will be transmitted through the reverse of the above process.
An extension of the hybrid system in Figure 2-18 illustrates how one of the miners is replaced by a nearby UHF leaky feeder cable, which acts as an extended antenna that is able to receive or transmit RF signals. The leaky feeder system would likely be the main communications system in the mine. The MF portion acts as an extension of the UHF leaky feeder, and the bridge repeater provides the conversion between the UHF leaky feeder and the MF system. Such a combination is one way to extend communications to the working face of the mine without having to extend the leaky feeder cable as the face advances.
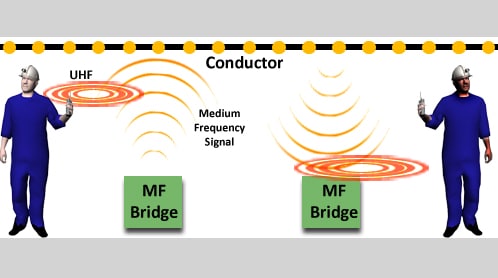
Figure 2-18. A Hybrid or Combination of MF/UHF Communications Systems
2.2.2.4 Through-the-Earth Systems
Through-the-earth (TTE) communications technology is the only technology that can transmit an electromagnetic signal between a sender and receiver with a worker underground and another on the surface without relying on a network or other additional infrastructure. Most electromagnetic waves normally reflect off the earth or rapidly weaken as they pass into the earth, such that they penetrate only a few feet below the surface. However, at frequencies less than about 10 kHz, it is possible for the waves to propagate more than 1,000 feet through the earth. There are several factors that limit potential applications for TTE in underground coal mines: antenna design, low frequencies necessary to transmit the signal, and other noise sources.
Antenna design has a large impact on TTE systems. An antenna is a metallic device that converts electromagnetic radiation into an electrical current; the variation in the electrical current carries the radio signal or signal information. When those currents flow on the antenna, they generate an electromagnetic wave. Similarly, if an electromagnetic wave impinges on an antenna, it generates currents on the antenna.
Antennas are most effective at transmitting and receiving RF wavelengths that are comparable to the largest dimensions of the antenna. This can be a problem for the very low frequency TTE waves. A 10-kHz electromagnetic wave has a wavelength in air of about 19 miles, which is not a practical size for an antenna. Thus, all practical TTE antennas are much smaller than a wavelength. The resulting inefficiency in the antenna means that only a very small fraction of the electromagnetic energy applied to the antenna radiates. Similarly, on reception, only a small portion of the electromagnetic energy contacting the antenna converts to an electrical signal on the wires connected to the antenna.
Loops or coils of wire are effective antennas for generating TTE electromagnetic waves, because they increase the surface area available for contact with the electromagnetic waves without increasing the overall footprint and it is relatively easy to lay out a loop in a room-and-pillar mine. For example, wrapping wires around a coal pillar creates a loop antenna. Figure 2-19 shows a representative TTE configuration - a loop on the surface transmitting a signal to a loop underground. To obtain a strong enough radio signal between the two loops, their coverage areas must partially overlap.
The low frequencies needed for TTE communications also limit the amount of information transmitted in the message and may delay the receipt of the message by several minutes. This limited information flow makes it very difficult to use TTE for voice communications and to include TTE communications links in a network.
Another limiting factor in the use of TTE communications is the variety of natural and man-made noise sources existing at these low frequencies, including electromagnetic energy from power lines and electromagnetic noise naturally occurring in the atmosphere. These noise sources further limit the range and information flow of a TTE communications link.
Given the constraints on antenna size, signal power, message size, and delivery delay, TTE systems are most likely to be used only in emergencies. An advantage of a TTE communications link is that it is highly survivable. As such, a TTE system could play a significant role as an emergency alternate communications path. Section 2.4 discusses alternate communications paths further.
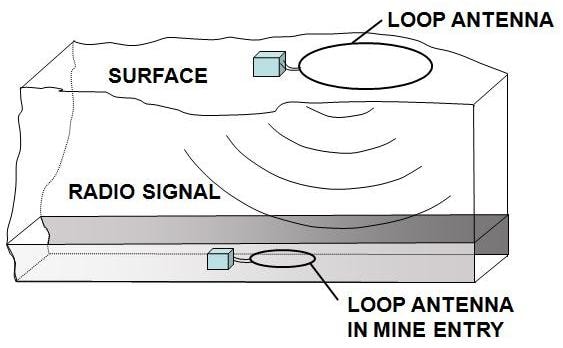
Figure 2-19. Example of a TTE Communications System
2.3 Tracking Systems Principles of Operation
2.3.1 Manual Tracking Systems
The intent of a tracking system is to record who is underground and where they are located. The mine operations center displays this information on the surface, so that in the event of an underground emergency, rescue workers can effectively plan their operations.
When using manual tracking, at the beginning of each shift, the mine foreman provides the dispatcher with a list of names of people and where they will be located in the mine. Once underground, if a miner needs to go to a different area to work, the miner notifies the dispatcher using the underground dial phone. The dispatcher then updates the list.
Manual tracking has a number of limitations. A miner may report a location as being within a working section, but that can be quite a large area, perhaps covering two square miles. Occasionally a miner will forget to notify the dispatcher when changing work locations. Also, the dispatcher has many duties and may not be available when a miner calls to notify the surface of a change in location. In an emergency, the phone system may not be operational.
Electronic tracking systems can address most, if not all, of these limitations. The following sections discuss several types of electronic systems.
2.3.2 Reader-based Tracking Systems
2.3.2.1 Radio Frequency Identification (Zone-Based)
Nearly all department stores have vertical sensor plates near their doors, which shoppers have to pass through on their way out of the store. If a customer has not paid for the merchandise, an alarm sounds. Radio Frequency Identification (RFID) is the basis for security systems such as this. Within the item being purchased is a small tag, typically about the size of a postage stamp, containing an electronic circuit. The vertical sensor plates at the exits are continuously emitting an RF signal. The sensor plates are RFID readers. If the reader signal reaches a tag (interrogates it), the tag sends back a response that is detected and read by the sensor plate and the alarm is sounded. On checkout, the tag is de-activated when the purchased item is rubbed on a particular region of the counter, producing a magnetic field that interacts with the circuit of the tag, making it inoperable.
The tags used in the department stores are passive tags, because the tag has no internal power source. Thus, the tag is passive and does not emit any RF energy until interrogated by the reader. Once interrogated, the tag absorbs a small amount of the RF energy from the interrogating signal and uses it to send a reply. Passive tags are very inexpensive because they are simple and have no internal battery. However, the range of the reader and tag system is quite short, typically a few feet; that is why the vertical readers at the store’s exit are so close together.
A more advanced level of RFID tracking can be used to track the locations of underground miners. To extend the tag-to-reader range, an active tag is used. Active tags have an internal battery to power the signal transmission. The tag is a very small radio, able to transmit and receive messages. Each miner wears a tag that transmits a unique identifier. Whenever the miner passes within the RF range of a reader, it interrogates the tag. The reader relays the detection information to a central location (usually the mine operations center) over wires, through fiber-optic cable, or even wirelessly. Each RFID reader has its own identification and a location associated with that identification. When a given reader interrogates a tag, then forwards the information to the operations center, personnel at the center know that the miner is within a certain distance of that reader’s location.
Figure 2-20 shows an example of RFID readers (blue circles) installed in a mine. Each reader has a location associated with a survey marker (black circles, each with a unique number). The blue ovals illustrate the RF range of each reader. The red ovals show the RF range of the miner’s tags. Miner A is within the RF range of the reader at survey marker 58301. Miner B is not within range of any reader, but if he had recently left the reader at marker 58289, his location would be his last recorded position. This is zone-based RFID because each reader only detects tags within its RF range or zone.
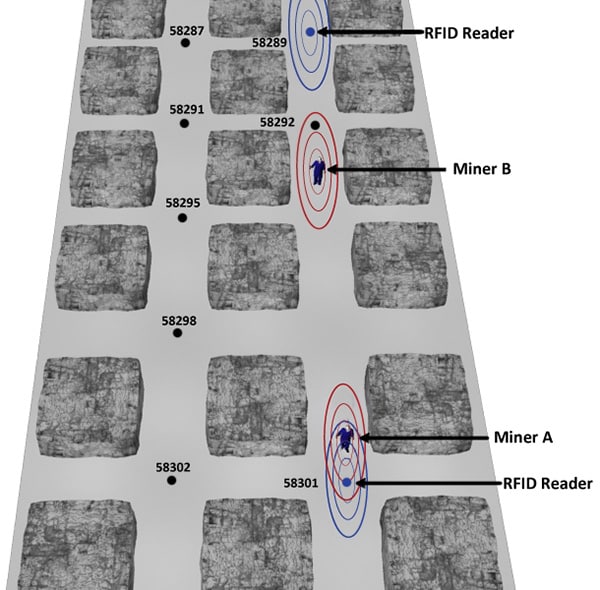
Figure 2-20. Zone-based RFID Tracking
The tracking system as presented in Figure 2-20 is independent of the communications system. Both the tracking and communications systems need to provide a link to the surface; therefore, it is logical to integrate the two systems. For example, the RFID readers could transmit their location information to a leaky feeder cable, which would then transfer the information to the operations center.
Not all RFID zone-based systems operate in the same way or use the same frequencies. Each manufacturer will develop features unique to their product. Given that flexibility, a representative RFID reader range is about 300 feet. In this case, the identified miner is within a circle with a radius of 300 feet (100 meters), centered at the RFID reader, as shown in Figure 2-21. Interpreting this figure allows us to determine possible locations for the miner. Clearly, the miner is not located within the coal; he is either in the entry with the reader or in one of the crosscuts. It is unlikely he is in a parallel entry (even though the 300-foot circle would permit it), because the RFID reader requires a line-of-sight with the tag (i.e., an unobstructed straight-line path between the tag and reader). Therefore, the miner must be within the red-shaded zone shown in Figure 2-21.
The resolution of a tracking system is a measure of the smallest detectable change in position or location of the miner. For RFID reader-based systems, a miner’s position is associated with a particular reader’s location and the resolution is determined by the reader spacing. Therefore, if a higher (i.e., better) resolution is required, more readers will be required, thus increasing the cost of the system.
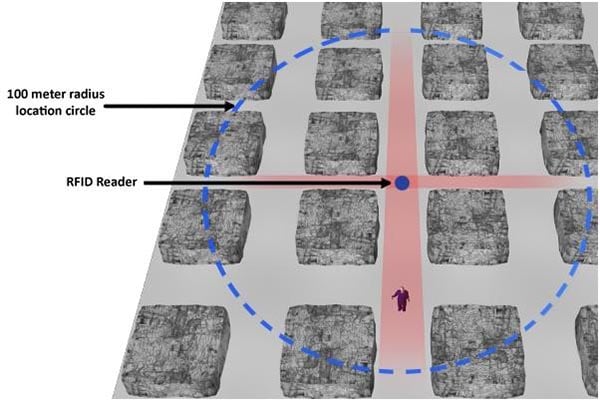
Figure 2-21. Miner Location with RFID Reader
2.3.2.2 Reverse RFID
Zone-based RFID systems are well established and are being used increasingly for industrial applications. However, for high-resolution applications over large areas these systems require a large number of readers, which in turn increases the cost and complexity. This section will discuss a relatively new approach, reverse RFID, which is currently being considered by some manufacturers.
In the reverse RFID system, each miner wears an RFID reader and the tags are in fixed, known locations. The location information obtained by the RFID reader must still reach the mine operations center. To accomplish this, the reader has a radio transmitter that periodically transmits the miner’s location data to the mine’s backhaul (the network’s backbone or the main route to the operations center) communications system. Figure 2-22 illustrates a reverse RFID system in which the backhaul is a UHF leaky feeder system.
The RFID tag periodically emits its identification information, shown in Figure 2-22. A separate antenna, possibly mounted on the miner’s cap, receives the RFID tag signal. The RFID information transfers to the transmitter on the miner’s belt, relays to the leaky feeder cable, and ultimately to the mine operations center. A UHF transmitter mounted on the miner’s belt transmits the location information to the leaky feeder cable.
RFID tags are relatively inexpensive when compared to the RFID reader. Tags can be located close together so that the miner’s location can be determined accurately. Each tag contains a battery, which makes maintenance a concern, but the batteries can last 10 years.
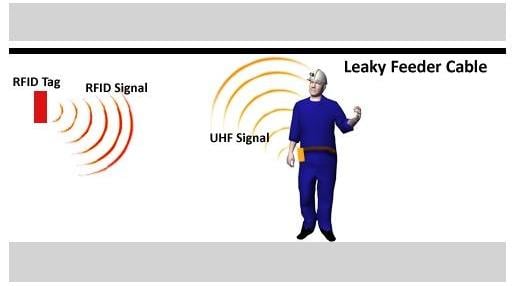
Figure 2-22. Example of Reverse RFID System with UHF Leaky Feeder Backhaul
Using a more sophisticated approach than just recognizing when a miner is in a certain zone can further enhance the location accuracy. If a miner is within RF range of two RFID tags at the same time, comparing the received signal strengths from the two tags can determine the miner’s location within 50 feet. As the miner approaches one tag, the strength of the signal from that tag increases. On the other hand, as the miner walks away from the other tag, the strength of the signal from that tag decreases. A comparison of the rates of change of signal strengths pinpoints the miner’s location. Analysis of this comparison also determines the miner’s speed and direction of travel. This technique is referred to as Received Signal Strength Indicator (RSSI).
2.3.3 Radio Node-Based Tracking Systems
Radio node-based tracking systems use the same physical components as the node-based communications systems. Radio node-based tracking uses the known locations of the fixed position nodes as reference points. Each handheld radio has a unique identifier assigned to it and the identifier is associated with a specific miner. A fixed node with a known location is linked to a radio with a unique ID and assigned to a specific miner, hence the location of the miner is known. Similar to RFID systems, the resolution is limited to the node spacing.
Applying the same concept of comparing radio signal strengths (RSSI), which is used in the reverse RFID technique, RSSI can be used for determining how far the miner’s radio, and thereby the miner, is from the node. In a reverse RFID system that uses RSSI, the tags are in fixed, known locations, and the miner wears a receiver that detects and measures the signals radiated by the tags. Since a node-based UHF communications system has all the necessary components to implement the RSSI technique, it does not require RFID tags. In a node-based system, the access node and/or the miner’s radio makes the signal strength measurements; hence the node based system provides both communications and tracking in a single system.
Positioning the nodes close enough so that there is continuous communications coverage allows the handheld radio to receive signals from multiple nodes and to determine the signal strengths. Each signal also contains information identifying the node from which it came. In some systems, the information accumulates in the miner’s radio and then transmits back to the mine operations center for analysis. Other systems compare the signal strength received from two or more nodes in the miner’s radio. The location is resolved using RSSI, which determines the distance of the miner from each of the nodes within the miner’s RF range.
Figure 2-23 illustrates the main features of a radio node-based tracking system. The red arcs represent the RF signals transmitted from the nodes. The illustration appears almost identical to the depiction of node-based communications as shown in Figures 2-14 through 2-16, which is why electronic tracking is easy to implement in a node-based communications system.
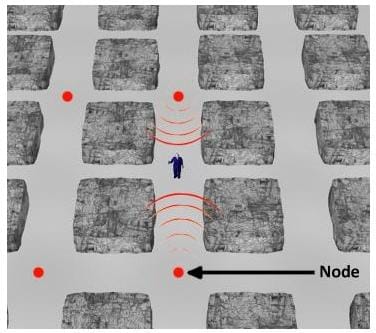
Figure 2-23. Radio Node-Based Tracking
Unfortunately, there are many factors in the underground mine environment that introduce uncertainties into the RSSI determination of location. These factors include any blockage that reduces or eliminates line-of-sight between the access node and the miner, including stoppings, equipment in the entries, turns in the entries, undulation in the coal seam, etc. With these uncertainties, the miner’s location may not be determined to better than about half the spacing of the distance between the nodes.
2.4 Network Options
Section 2.1.2 introduced the concept of networks. A communications network is an interconnection of communications components that allow a user to send a message to a specified destination. The network receives, interprets, transports, and delivers the message to the destination. For simplicity, this discussion focuses on communications networks, but it applies equally well to electronic tracking networks.
Figure 2-24 illustrates the main responsibilities of a network - access and transport. The concepts of access and transport are analogous to a city bus network. The buses travel predefined routes and pick up riders at specified locations; but to achieve transport, a rider must access the system (go to a bus stop). Similarly, in a communications or tracking network, the interconnected nodes provide the message transport, but the user must access the network in order to send the message.
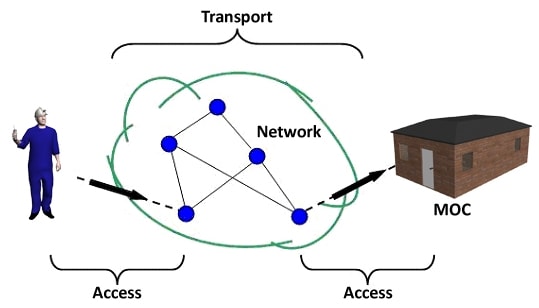
Figure 2-24. Main Responsibilities of a Network: Access and Transport
Section 2.2.2.1 on leaky feeders introduced the idea of an alternate communications path. Alternate communications paths provide the key to ensuring the survivability of systems in a coal mine, and the support of these alternate communications paths is a critical consideration in evaluating a mine operator’s network option.
Figure 2-25 shows a leaky feeder system, which establishes redundancy by providing an alternate communications path by means of an overland, fiber-optic link between the airshaft and the primary base station at the elevator shaft. The surface link maintains the communications even if there is damage to the underground connection between the two shafts. Figure 2-25 introduces the issue that an alternate communications path typically emerges at some point on the surface separated, possibly by miles, from the primary path exit point. In this example, the primary base station would generally be located at the mine operations center (MOC) and a mechanism is required to connect the secondary base station back to the MOC. As shown in Figure 2-25, the overland connection could be fiber-optic cable. Other options include hardwiring through leased lines from the telephone company, or a wireless link.
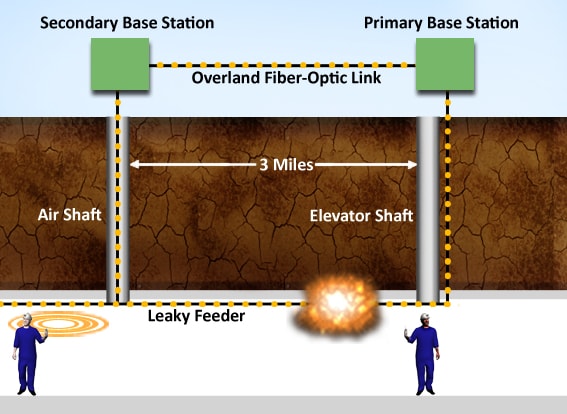
Figure 2-25. Example of an Alternate Communications Path
2.5 Mine Operations Center
The MINER Act requires wireless communications between underground miners and surface personnel. The MINER ACT also requires the use of electronic tracking systems by personnel on the surface for obtaining information on the location of underground workers. Both of these requirements are administered through the mine operations center, the central location of a mine’s operations on the surface above the mine.
2.5.1 Tracking Displays
The MINER Act specifies that surface personnel must have the current location or immediately pre-accident location of all underground miners. There are a number of ways that tracking systems display the location of miners. One method is a simple list of the names or identifying employee numbers of all miners underground along with the nearest survey spad station. An easier format to interpret is a computer display of a mine map with the names of the personnel working underground. Zoom and pan features would make it possible to view the entire underground mine, including the current location of all personnel. Figure 2-26 shows a sample display.
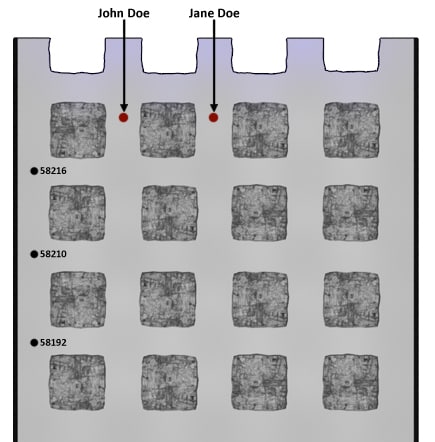
Figure 2-26. Sample Display of Miner Locations
2.5.2 Surface Communications
The communications network established underground must provide access to surface personnel. As discussed in Section 2.4, there may be multiple points where the alternate communications paths exit the mine to the surface. These locations are referred to as surface points of presence (POPs). Each of these surface POPs should be linked, or be able to be linked in the case of an emergency, to the mine operations center.
There are a variety of options available for linking these POPs back to the operations center. Where the mine operator has access to the required real estate and surface rights, the operator could install fiber-optic cable, wires, or a wireless link directly back to the mine operations center. Another option is to lease copper lines from the telephone company. These lines appear to the mine operator as a directly connected line to the POPs and the operator can connect his communications right into the lines.
A third option, with widespread availability due to the proliferation of Internet Protocol (IP) capable devices and “broadband” networks, is to lease a connection that relies on the Internet. This option includes DSL modems through the telephone companies, cable modems through cable television companies, and satellite modems through satellite service providers. While not all mine communications inherently support IP protocols, the equipment vendors are beginning to offer such an option; currently, converters that enable mine communications through the Internet are available.
An advantage of using the Internet-based approach is that mines can remotely monitor their communications links and other systems within the mine. Some equipment vendors and third-party providers already offer services to monitor the systems, thus eliminating the burden of the mine operations personnel of monitoring and troubleshooting the networks.
Because mining communications systems are for day-to-day routine use as well as for emergencies, when an emergency occurs, it is essential that a protocol exists to ensure that the dispatcher in the mine operations center immediately recognizes when the nature of the communication is an emergency. An emergency communication generally includes audio alarms for voice communications systems or visual alarms that display on the screen for text systems.
3.0 Acronyms
CT
DAS
EM
ERP
Hz
IP
MF
RF
RFID
RSSI
TTE
UHF
VHF
WLAN
communications and electronic tracking
distributed antenna system
electromagnetic
emergency response plan
hertz
internet protocol
medium frequency
radio frequency
radio frequency identification
received signal strength indicator
through the earth
ultra high frequency
very high frequency
wireless local area network
- Advanced Tutorial on Wireless Communication and Electronic Tracking: Communication System Performance
- Advanced Tutorial on Wireless Communication and Electronic Tracking: Introduction
- Assessment of Present Electromagnetic Techniques for the Location of Trapped Miners
- Emergency Communications and Tracking
- Experience with an Integrated, Computer-Controlled Communications and Monitoring System at the Robena Mine
- Mine Communications Engineering and Compatibility Guidelines
- Propagation of EM Signals in Underground Metal/Non-Metal Mines
- Propagation of EM Signals in Underground Mines
- Subterranean Wireless Electronic Communication System
- Underground Mine Communications
- Wireless Through-The-Earth Modeling and Support